Current and emerging radiotherapy strategies for pancreatic adenocarcinoma: stereotactic, intensity modulated and particle radiotherapy
Introduction
Pancreatic ductal adenocarcinoma (PDAC) is projected to be the 3rd leading cause of cancer-related deaths in the United States with an estimated 5-year overall survival (OS) of approximately 8% (1). Surgery is the only curative approach, but the majority of patients tend to present with advanced stage of disease. In a patient with unresectable or locally advanced pancreatic cancer (LAPC), radiotherapy with or without chemotherapy remains an important modality in achieving local control. Recent results of the LAP07 study that compared chemotherapy alone vs. chemoradiation for LAPC demonstrated no difference in OS, but a significantly better local control for the chemoradiation arm (2). This study has brought the role of radiation in pancreatic cancer into question, and a selective approach to its use is favored. Furthermore, conventional fractionation delivered over 6 weeks was utilized in the majority of studies for LAPC. This approach with a long course of chemoradiation has a long track record, but conventional doses are still sub-therapeutic, achieving local control rates of 70–80% at 2 years. Therefore, there is increasing interest to explore other radiotherapy techniques or regimens to improve patients’ outcomes with LAPC. Here we review the literature and summarize the strategies for utilizing modern and emerging radiation treatment techniques for patients with LAPC.
Stereotactic body radiotherapy (SBRT) for LAPC
SBRT is an advanced form of radiation therapy that enables the delivery of high radiation doses per fraction to the tumor target while having a rapid dose gradient falloff beyond the target (Figure 1). This characteristic of SBRT limits the dose to the surrounding normal tissues. SBRT treatment usually utilizes hypofractionation, meaning that doses per fraction are higher than conventional treatments (>2 Gy per fraction) and delivered in fewer fractions. This achieves similar equivalent total dose as a conventional fractionation. Assuming an alpha/beta of 10, the EQD2 for SBRT is 45.6 Gy (6.6 Gy per fraction for 5 fractions), as compared to 50 Gy for conventional fractionation (50 Gy in 25 fractions). The higher dose per fraction for SBRT may theoretically produce greater biological cell kill (3), but clinical studies suggest similar pathological response rates as conventional fractionation (4). One of the practical advantages of SBRT over conventional chemoradiation is the shorter time of delivery, reducing the duration to be off systemic treatment.
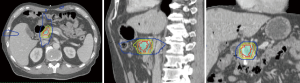
Partly due to the convenience of finishing radiation within 1 week and the increasing utilization of technology in radiation practice, the interest in SBRT for PDAC has increased. The goal is to deliver a high dose to the tumor that is safe, respecting the dose tolerance of surrounding normal structures such as the small bowel and stomach.
One of the initial approaches to SBRT involved a single dose of radiation to the tumor. Investigators at Stanford reported the feasibility and safety of single dose SBRT in 6 patients with LAPC (5). However, a subsequent larger SBRT study showed that although single dose SBRT was efficacious, the rate of late toxicity was high with up to 20% of Grade 2+ toxicity (6). Multiple other publications, mostly retrospective studies, have reported the efficacy and toxicity of SBRT in patients with LAPC (Table 1). Overall, fractionated SBRT regimens of 3 to 5 fractions are as efficacious as single fraction treatment achieving a 1-year local control of approximately 80% but fractionated treatments are better tolerated than single fraction SBRT.
Table 1
Study | Nature of study and number of patients | Dose/fractionation | Outcomes | Acute toxicity (≥ Grade 3) | Toxicity (≥ Grade 3) |
---|---|---|---|---|---|
Chang et al., 2009 (7) | Retrospective, 77 (LAPC) | 25 Gy/1 fraction | 1-year FFLP: 84%; 1-year PFS: 9%; 1-year OS: 21% | Gastric ulcer [1] | Gastric ulcer [3], duodenal stricture [1], biliary stricture [2] |
Chuong et al., 2013 (8) | Retrospective, 73 (LAPC and borderline) | 25 Gy/5 fractions | 1-year PFS: 42.8% (borderline), 41% (LAPC); |
GI bleeding [3], anorexia [1] | |
Comito et al., 2017 (9) | Phase II, 45 (LAPC) | 45 Gy/6 fractions | 2-year FFLP: 90%; |
None | |
Gurka et al., 2017 (10) | Retrospective 38 (LAPC and borderline) | 25–30 Gy/5 fractions | 6-month FFLP: 82%; |
Gastric outlet obstruction [1], biliary obstruction [1], GI bleeding (1–Grade 5) | |
Herman et al., 2015 (11) | Phase II 49 (LAPC) | 33 Gy/5 fractions | 1-year FFLP: 78%; |
Duodenal ulcer [1], |
Fistula [1], ulcer [3] |
Mahadevan et al., 2011 (12) | Retrospective 39 (LAPC) | 24–36 Gy/3 fractions | Local control 85% (median follow-up: 21 months); |
GI bleeding [2], gastric outlet obstruction [1] | |
Mellon et al., 2015 (13) | Retrospective 159 (LAPC and borderline) | 28–30 Gy/5 fractions | Median OS: 19.2 months (borderline), 15 months (LAPC) | GI bleeding [6] | |
Pollom et al., 2014 (14) | Retrospective 167 (LAPC) | 25 Gy/1 fraction [76]; 25–45 Gy/5 fraction [91] | 1-year OS: 33.1% | GI bleed [1], gastric ulcer [1] | Duodenal perforation [3], biliary stricture [1], gastric ulcer [4], GI bleed [1], duodenal ulcer [2], duodenal stricture [2] |
Schellenberg et al., 2008 (6) | Phase II 16 (LAPC) | 25 Gy/1 fraction | 1-year OS: 50%; median OS: 11.4 months | Gastric outlet obstruction [1] | Acute: gastric outlet obstruction [1] |
Late: duodenal perforation [1], duodenal stenosis [1] | |||||
Tozzi et al., 2013 (15) | Prospective, 30, (21 LAPC +9 recurrence post-surgery) | 45 Gy/6 fractions | 1-year FFLP: 77%; 2-year FFLP: 77%; median PFS: 8 months; 1-year OS: 47% | None | |
Rwigema et al., 2011 (16) | Retrospective 71 (40 LAPC, 11 recurrence post surgery, 8 with metastatic disease, 12 adjuvant treatment) | 18–25 Gy/1–4 fractions | 1-year FFLP: 48.5% (73% in those who received ≥24 Gy vs. 45% in those who had lower doses); |
Nausea [1], enteritis [1], gastroparesis [1] | None |
IMRT, intensity modulated radiation therapy; SBRT, stereotactic body radiotherapy; LAPC, locally advanced pancreatic cancer; OS, overall survival; PFS, progression-free survival; FFLP, freedom from local progression; GI, gastrointestinal.
Achieving these results with SBRT requires error margins less than 2 mm, in general. This degree of accuracy can be attained with on-board imaging such as cone-beam CT or CT-on-rails (5,17,18), in combination with respiratory motion management with breath hold or abdominal compression (17,19). Finally, to achieve sharp dose gradients, intensity modulated radiation therapy is used. With these technologies, SBRT demonstrates low rates of Grade 3 or higher radiation-related late toxicities (Tables 1,2); however, some toxicities such as gastrointestinal bleeding and perforation can be fatal. Therefore, proper quality assurance of treatment planning and delivery is essential to minimize the risk of such complications.
Table 2
Effects | IMRT | SBRT | Proton |
---|---|---|---|
Acute effects | |||
Nausea/vomiting | ++ | + | ++ |
Diarrhoea | + | − | + |
Dermatitis | − | − | + |
Late effects | |||
GI ulcer/bleed | + | ++ | + |
GI obstruction | ++ | + | − |
Bile duct stenosis | + (in dose escalated regimen) | + | Unknown |
IMRT, intensity modulated radiation therapy; SBRT, stereotactic body radiotherapy; GI, gastrointestinal.
Intensity modulated radiation therapy (IMRT) for LAPC (Table 3)
Table 3
Study | Nature of study and number of patients | Dose/fractionation | Concurrent chemotherapy | Outcomes | Acute toxicity (≥ Grade 3) | Late toxicity (≥ Grade 3) |
---|---|---|---|---|---|---|
Abelson et al., 2012 (20) | Retrospective, 47 (IMRT; 29 adjuvant, 18 definitive) | 50.4 Gy/28 fractions for adjuvant cases; 54 Gy/30 fractions for definitive cases | Capecitabine /5-FU | 1-year FFLP: 92%; 2-year FFLP: 80%; 1-year OS: 79%; 2-year OS: 40% | Biliary stent blockage [1], cholangitis [1], small bowel obstruction [1], enteritis (1, died) | Small bowel obstruction [1], |
Colbert et al., 2017 (21) | Retrospective, 154 (59 IMRT, 95 3D-CRT) | For 3D-CRT: 50.4 Gy/28 fractions | Gemcitabine/5-FU/cetuximab | IMRT group (dose escalation): anorexia [2]; rate of acute Grade 3 toxicities were lower in IMRT (dose-escalated) than 3D-CRT (standard dose): 4% vs. 16% (P=0.004) | IMRT group (dose escalation): |
|
For IMRT: all had BED >70 Gy (63 Gy/28 fractions, 67.5 Gy/15 fractions, 70 Gy/28 fractions) | ||||||
Krishnan et al., 2016 (22) | Retrospective, 200 (all LAPC); Dose escalation group: 41 IMRT, 5 3D-CRT with IMRT boost, 1 IMRT with proton therapy boost); Standard dose group: 13 IMRT, 140 3D-CRT) | Standard dose group: 50.4 Gy/28 fractions or 50 Gy/25 fractions | Capecitabine/5-FU | Median FFLP: 7.3 months; |
Dose-escalated group: Diarrhoea [1], GI bleeding [1] | |
Dose-escalated group: 57.25 Gy in 25 fractions | Those with BED >70 Gy had improved outcomes than those with BED ≤70 Gy | |||||
Prasad et al., 2016 (23) | Retrospective, 205 (134 IMRT, 71 3D-CRT) | 50.4 Gy/28 fractions; IMRT cases: simultaneous boost to gross disease to 56 Gy | Gemcitabine +/− 5-FU/capecitabine | 3D-CRT: Diarrhoea [2], Nausea [3]; IMRT: Diarrhoea [3] | ||
Yovino et al., 2011 (24) | Retrospective, 46 IMRT cases; toxicities compared to toxicity data from 100 patients from RTOG97-04 (3D-CRT) | 50.4 Gy/28 fractions | Capecitabine/5-FU | Median PFS: 17.5 months; |
IMRT: Diarrhoea [2], anorexia [2]; 3D-CRT (RTOG97-04): Diarrhoea [78], nausea/vomiting [49], anorexia [9] | IMRT: small bowel obstruction [2] |
IMRT, intensity modulated radiation therapy; SBRT, stereotactic body radiotherapy; LAPC, locally advanced pancreatic cancer; OS, overall survival; GI, gastrointestinal; PFS, progression-free survival; BED, biological equivalent dose; FFLP, freedom from local progression; 3D-CRT, 3D conformal radiation technique.
IMRT is a well-established contemporary radiation planning and delivery technique which utilizes multiple radiation beams to delivery conformal dose to the target allowing sparing of adjacent normal organs. Prior to the implementation or advancement of IMRT technique, 3D conformal radiation technique (3D-CRT) was used. In 3D-CRT, typically 3 to 4 beams were used to deliver dose to the target (the intersection of all beams). In IMRT, typically 7 to 9 beams are used to allow a more conformal dose delivery to the target while spreading the low dose scatter across the beams. While this IMRT approach generally achieves lower maximal doses to the surrounding normal organs than 3D-CRT, it results in a larger volume of the body receiving a low dose bath (i.e., higher integral dose).
There has been no prospective comparative study to show that IMRT is better tolerated than 3D-CRT. In a systematic review by Bittner et al. (25), the authors collated treatment-related toxicities from 13 IMRT studies and seven 3D-CRT studies. Although no substantial difference in survival was observed between the two treatment techniques, the authors reported Grade 3 or higher acute gastrointestinal toxicities were significantly lower in those who had IMRT (7.8% vs. 13.4%, P<0.001 for nausea and vomiting, and 2% vs. 11.6%, P<0.001 for diarrhea). In addition, and more importantly, there was significantly less Grade 3 or higher late toxicity in those who had IMRT compared to 3D-CRT (5% vs. 10.6%, P=0.017). A retrospective study by Colbert et al. (21) showed that patients who had IMRT to a higher dose (escalated dose fractionation) had lower rates of Grade 3+ acute toxicities during radiotherapy, compared to those who had 3D-CRT with conventional dose fractionation of 50.4 Gy in 28 fractions.
The ability to “dose paint” is one main advantage of IMRT over 3D-CRT. IMRT may be used in the context of SBRT (delivery within 3–6 fractions) or longer courses of radiation (15–28 fractions). The use of IMRT over longer courses of radiation has been studied for conventional doses of radiation treatment (50 Gy in 1.8 to 2 Gy per fraction) (Figure 2) and escalated doses (Figure 3). The conventional radiation dose provided relatively good local control (~70–80% at 2 years) but randomized studies have not been shown to improve OS in patients with LAPC. Historically, the standard dose was set at 50.4 Gy due to the dose tolerance of adjacent normal structures such as small bowel and the stomach. However, with IMRT, the total dose to the tumor can be increased while ensuring the dose constraints of normal tissues are respected. The MD Anderson Cancer Center group had showed improved outcomes in terms of OS and locoregional relapse free survival in patients who received escalated dose (biological equivalent dose >70 Gy) compared to those who had standard radiation dose or biological equivalent does of <70 Gy (22). Only 1 out of 200 patients in the cohort developed Grade 3 acute toxicity. In a follow-up comparative study of treatment-related toxicities in patients who had a dose escalated regimen (biological equivalent dose >70 Gy) using IMRT and those who had standard radiation dose of 50.4 Gy in 28 fractions delivered using 3D-CRT, the group observed an overall lower rates of Grade 3 acute in those who received dose-escalated regimen delivered using IMRT compared those who received standard radiation dose delivered using 3D-CRT technique (4% vs. 16%, P=0.004) (21). Six of 59 patients who received the dose-escalated regimen developed Grade 3+ late toxicity in form of either gastrointestinal stricture or bleeding. Therefore, with current advancements in imaging during radiotherapy, dose-escalated radiation therapy either using IMRT or SBRT is an area of interest for further investigation.
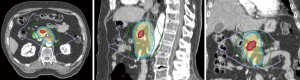
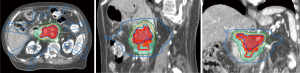
Proton and carbon ion therapy for LAPC (Table 4)
Table 4
Study | Nature of study and number of patients | Dose/fractionation | Concurrent chemotherapy | Outcomes | Acute toxicity (≥ Grade 3) | Late toxicity (≥ Grade 3) |
---|---|---|---|---|---|---|
Hong et al., 2014 (26) (Proton) | Prospective (Neoadjuvant), 50 | 25 Gy/5 fractions | Capecitabine | 11 patients did not have surgery | Colitis [1], chest wall pain [1] | |
Out of 48 patients: PFS: 10.4 months; OS: 17.3 months; 2-year OS: 42%; | ||||||
Out of 37 patients who had surgery: PFS: 14.5 months; OS: 27 months | ||||||
Nichols et al., 2013 (27) (Proton) | Retrospective, 22 [5 resectable, 5 borderline resectable (BRPC), 12 LAPC] | 50.4–59.4 Gy/28–33 fractions | Capecitabine | Median survival: Resectable—11 months; BRPC—14 months; LAPC—8.8 months | None | |
Sachsman et al., 2014 (28) (Proton) | Prospective (Definitive), 11 | 59.4 Gy/33 fractions | Capecitabine | 2-year PFS: 14%; OS: 18.4 months; 2-year OS: 31%; 2-year FFLP: 69% | None | |
Terashima et al., 2012 (29) (Proton) | Prospective (Definitive), 50 | P1: 50 Gy/25 fractions [5]; P2: 70.2 Gy/26 fractions [5]; P3: 67.5 Gy/25 fractions [40] | Gemcitabine | Overall: 1-year PFS: 64.3%; 1-year OS: 76.8%; 1-year FFLP: 81.7%; | P1 and P2: acute GI bleeding [1] | P3: GI ulcer treated with medications [3]; death from GI bleed [1] |
P3 patients: 1-year PFS: 60.8%; 1-year OS: 78.8%; 1-year FFLP: 79.9% | ||||||
Ito et al., 2004(30) (Carbon) | Retrospective (Neoadjuvant), 4 | 48 GyE (covering pancreas, lymph nodes and nervous plexus) | Post-operative cholangitis [1] | None | ||
Shinoto et al., 2016 (31) (Carbon) | Prospective (Definitive), 71 | 43.2–55.2 GyE/12 fractions | Gemcitabine | 1-year OS: 73%; 2-year OS: 35%; Median OS: 19.6 months | Anorexia [6], GI bleed [1] | |
Okada et al., 2010 (32) (Carbon) | Prospective (Neoadjuvant), 22 | 16 fractions over 4 weeks | 1-year FFLP: 100%; 2-year FFLP: 87%; 2-year OS: 24% (7 patients did not proceed to surgery) | |||
Prospective (Definitive), 31 | 38.4–48 GyE/12 fractions/3 weeks | 1-year FFLP: 81%; 1-year OS: 44% | None | None |
IMRT, intensity modulated radiation therapy; SBRT, stereotactic body radiotherapy; LAPC, locally advanced pancreatic cancer; BRPC, borderline resectable pancreatic cancer; OS, overall survival; GI, gastrointestinal; PFS, progression-free survival; BED, biological equivalent dose; FFLP, freedom from local progression.
Proton therapy (Figure 4) is an emerging radiation modality that is of increasing interest due to its unique properties of having no exit dose in beam path beyond the target (33). Radiobiologically, protons, as positively charged particles, have higher linear energy transfer (LET) compared to photons (currently utilized in SBRT and IMRT), thus translating to potentially greater double-stranded DNA damage in targeted region and higher cell kill than photon. Although the idea of using proton therapy in patients with LAPC is attractive due to the potential benefits of greater cell kill and possibly minimal acute and late toxicity due to less low dose scatter, the use of proton therapy in treating patients with pancreatic cancer is currently investigational and the clinical evidence is limited (Table 4).
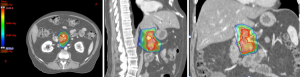
The dosimetric benefit of proton therapy compared to IMRT was demonstrated in a study by Thompson et al. (34). Using planning images from 13 patients with unresectable pancreatic cancer, they showed that proton plans had significantly lower stomach and small bowel doses than IMRT plans in the 20–45 Gy regions. Furthermore, there was 50% less mean liver dose and 18% less mean kidney dose in the generated proton plans compared to IMRT plans. Within the intermediate to high dose regions of at least 45 Gy, the proton plans had higher dose than IMRT plans. Therefore, this study suggests that the treating physician should be mindful of the radiation ‘hot spots’ within the intermediate to high dose regions when reviewing a proton plan. However, the ‘hot spot’ generated by protons may be exploited if it is deposited within the tumor, thereby delivering an escalated dose to the tumor and generating greater cell kill.
Hong and colleagues (26), in a prospective phase I/II trial, demonstrated the feasibility and safety of short course (25 Gy in 5 fractions) proton therapy in 50 patients with borderline resectable pancreatic cancer. Forty-eight patients were evaluable. Only two patients developed Grade 3+ acute toxicity—one with colitis, and another with chest wall pain. Eleven patients did not have subsequent surgical resection. The cohort, as a whole, achieved a median progression-free and OS of 10 and 17 months, and a 2-year OS of 42%. In those who had surgery, they achieved a median progression-free and OS of 15 and 27 months. In another prospective study of 11 patients by Sachsman et al. (28) using a conventional fractionated regimen (59.4 Gy in 33 fractions) demonstrated a 2-year freedom from local progression rate of 69% and 2-year OS rate of 31%. No significant late effects were reported.
Although the evidence for proton therapy is limited, the above 2 studies have established that proton therapy is feasible, and can be further explored as a modality of treatment for patients with pancreatic cancer. The biological effects of protons to normal tissues as compared to photons remain to be accurately determined, and on-board imaging of proton therapy for pancreatic cancer treatments is yet to be optimized. Therefore, this modality should be used with caution and further guidelines with regards to patient selection, dose/fractionation, on-board imaging, and internal organ motion management during proton therapy are required.
Another form of particle-based therapy is carbon ions. This therapy is currently being explored in pancreatic cancer treatment. There is no carbon ion facility in North America currently. In Japan, investigators have reported their experience in treating patients with pancreatic cancer with carbon ions, showing promising outcomes with minimal acute toxicities (30,32,35) (Table 4). There has been no data on the potential late toxicities of carbon ion therapy as yet.
Conclusions
The current data for PDAC suggest that a selective approach should be used when conventional radiation therapy is delivered (50 Gy in 25–28 fractions). In the era of personalized therapy, there are emerging and continuing efforts to improve radiation treatment and outcomes for patients with PDAC. As the tumor is frequently located in close proximity to radiosensitive gastrointestinal structures, advancements in treatment techniques and imaging modalities have enabled the effective and safe delivery of higher doses of radiation, and there is evidence that these higher doses may translate to better outcomes. Further study is warranted with these emerging techniques of SBRT, escalated dose radiation with IMRT, and charged particle radiation.
Acknowledgments
Funding: We gratefully acknowledge support from the Andrew Sabin Family Fellowship, the Sheikh Ahmed Center for Pancreatic Cancer Research, institutional funds from The University of Texas MD Anderson Cancer Center Moonshots Program, GE Healthcare, Philips Healthcare, Project Purple, and Cancer Center Support (Core) Grant CA016672 from the National Cancer Institute to MD Anderson. Dr. Eugene Koay was also supported by NIH (U54CA210181-01, U01CA200468 and U01CA196403), the Pancreatic Cancer Action Network (14-20-25-KOAY), and the Radiological Society of North America (RSD1429). Dr. Sweet Ping Ng was also supported by the Australian Postgraduate Award, the Radiological Society of North America Fellow Grant, and the Royal Australian and New Zealand College of Radiologists Research Grant.
Footnote
Provenance and Peer Review: This article was commissioned by the Guest Editor (Richard Tuli) for the series “Radiotherapy and Pancreatic Adenocarcinoma” published in Annals of Pancreatic Cancer. The article has undergone external peer review.
Conflicts of Interest: Both authors have completed the ICMJE uniform disclosure form (available at http://dx.doi.org/10.21037/apc.2018.07.03). The series “Radiotherapy and Pancreatic Adenocarcinoma” was commissioned by the editorial office without any funding or sponsorship. SPN reports grants from RSNA, grants from RANZCR grants, other from Australian postgraduate award, outside the submitted work. EJK reports grants and personal fees from University of Texas MD Anderson Cancer Center, grants from National Cancer Institute, grants from Philips, GE, Elekta, Stand up to cancer, Project Purple, outside the submitted work; In addition, EJK has a patent Apparatus and Methods for Three-Dimensional Printed Oral Stents for Head and Neck Radiotherapy pending and Radiation Oncology representative for Hepatobiliary Task Force, NCI. The authors have no other conflicts of interest to declare.
Ethical Statement: The authors are accountable for all aspects of the work in ensuring that questions related to the accuracy or integrity of any part of the work are appropriately investigated and resolved.
Open Access Statement: This is an Open Access article distributed in accordance with the Creative Commons Attribution-NonCommercial-NoDerivs 4.0 International License (CC BY-NC-ND 4.0), which permits the non-commercial replication and distribution of the article with the strict proviso that no changes or edits are made and the original work is properly cited (including links to both the formal publication through the relevant DOI and the license). See: https://creativecommons.org/licenses/by-nc-nd/4.0/.
References
- Siegel RL, Miller KD, Jemal A. Cancer Statistics, 2017. CA Cancer J Clin 2017;67:7-30. [Crossref] [PubMed]
- Hammel P, Huguet F, van Laethem JL, et al. Effect of Chemoradiotherapy vs Chemotherapy on Survival in Patients With Locally Advanced Pancreatic Cancer Controlled After 4 Months of Gemcitabine With or Without Erlotinib: The LAP07 Randomized Clinical Trial. JAMA 2016;315:1844-53. [Crossref] [PubMed]
- Stauder MC, Miller RC. Stereotactic Body Radiation Therapy (SBRT) for Unresectable Pancreatic Carcinoma. Cancers (Basel) 2010;2:1565-75. [Crossref] [PubMed]
- Rajagopalan MS, Heron DE, Wegner RE, et al. Pathologic response with neoadjuvant chemotherapy and stereotactic body radiotherapy for borderline resectable and locally-advanced pancreatic cancer. Radiat Oncol 2013;8:254. [Crossref] [PubMed]
- Koong AC, Christofferson E, Le QT, et al. Phase II study to assess the efficacy of conventionally fractionated radiotherapy followed by a stereotactic radiosurgery boost in patients with locally advanced pancreatic cancer. Int J Radiat Oncol Biol Phys 2005;63:320-3. [Crossref] [PubMed]
- Schellenberg D, Goodman KA, Lee F, et al. Gemcitabine chemotherapy and single-fraction stereotactic body radiotherapy for locally advanced pancreatic cancer. Int J Radiat Oncol Biol Phys 2008;72:678-86. [Crossref] [PubMed]
- Chang DT, Schellenberg D, Shen J, et al. Stereotactic radiotherapy for unresectable adenocarcinoma of the pancreas. Cancer 2009;115:665-72. [Crossref] [PubMed]
- Chuong MD, Springett GM, Freilich JM, et al. Stereotactic body radiation therapy for locally advanced and borderline resectable pancreatic cancer is effective and well tolerated. Int J Radiat Oncol Biol Phys 2013;86:516-22. [Crossref] [PubMed]
- Comito T, Cozzi L, Clerici E, et al. Can Stereotactic Body Radiation Therapy Be a Viable and Efficient Therapeutic Option for Unresectable Locally Advanced Pancreatic Adenocarcinoma? Results of a Phase 2 Study. Technol Cancer Res Treat 2017;16:295-301. [Crossref] [PubMed]
- Gurka MK, Kim C, He AR, et al. Stereotactic Body Radiation Therapy (SBRT) Combined With Chemotherapy for Unresected Pancreatic Adenocarcinoma. Am J Clin Oncol 2017;40:152-7. [Crossref] [PubMed]
- Herman JM, Chang DT, Goodman KA, et al. Phase 2 multi-institutional trial evaluating gemcitabine and stereotactic body radiotherapy for patients with locally advanced unresectable pancreatic adenocarcinoma. Cancer 2015;121:1128-37. [Crossref] [PubMed]
- Mahadevan A, Miksad R, Goldstein M, et al. Induction gemcitabine and stereotactic body radiotherapy for locally advanced nonmetastatic pancreas cancer. Int J Radiat Oncol Biol Phys 2011;81:e615-22. [Crossref] [PubMed]
- Mellon EA, Hoffe SE, Springett GM, et al. Long-term outcomes of induction chemotherapy and neoadjuvant stereotactic body radiotherapy for borderline resectable and locally advanced pancreatic adenocarcinoma. Acta Oncol 2015;54:979-85. [Crossref] [PubMed]
- Pollom EL, Alagappan M, von Eyben R, et al. Single- versus multifraction stereotactic body radiation therapy for pancreatic adenocarcinoma: outcomes and toxicity. Int J Radiat Oncol Biol Phys 2014;90:918-25. [Crossref] [PubMed]
- Tozzi A, Comito T, Alongi F, et al. SBRT in unresectable advanced pancreatic cancer: preliminary results of a mono-institutional experience. Radiat Oncol 2013;8:148. [Crossref] [PubMed]
- Rwigema JC, Parikh SD, Heron DE, et al. Stereotactic body radiotherapy in the treatment of advanced adenocarcinoma of the pancreas. Am J Clin Oncol 2011;34:63-9. [Crossref] [PubMed]
- Crane CH. Hypofractionated ablative radiotherapy for locally advanced pancreatic cancer. J Radiat Res 2016;57:i53-i7. [Crossref] [PubMed]
- Koay EJ, Hall W, Park PC, et al. The role of imaging in the clinical practice of radiation oncology for pancreatic cancer. Abdom Radiol (NY) 2018;43:393-403. [Crossref] [PubMed]
- Abbas H, Chang B, Chen ZJ. Motion management in gastrointestinal cancers. J Gastrointest Oncol 2014;5:223-35. [PubMed]
- Abelson JA, Murphy JD, Minn AY, et al. Intensity-modulated radiotherapy for pancreatic adenocarcinoma. Int J Radiat Oncol Biol Phys 2012;82:e595-601. [Crossref] [PubMed]
- Colbert LE, Moningi S, Chadha A, et al. Dose escalation with an IMRT technique in 15 to 28 fractions is better tolerated than standard doses of 3DCRT for LAPC. Adv Radiat Oncol 2017;2:403-15. [Crossref] [PubMed]
- Krishnan S, Chadha AS, Suh Y, et al. Focal Radiation Therapy Dose Escalation Improves Overall Survival in Locally Advanced Pancreatic Cancer Patients Receiving Induction Chemotherapy and Consolidative Chemoradiation. Int J Radiat Oncol Biol Phys 2016;94:755-65. [Crossref] [PubMed]
- Prasad S, Cambridge L, Huguet F, et al. Intensity modulated radiation therapy reduces gastrointestinal toxicity in locally advanced pancreas cancer. Pract Radiat Oncol 2016;6:78-85. [Crossref] [PubMed]
- Yovino S, Poppe M, Jabbour S, et al. Intensity-modulated radiation therapy significantly improves acute gastrointestinal toxicity in pancreatic and ampullary cancers. Int J Radiat Oncol Biol Phys 2011;79:158-62. [Crossref] [PubMed]
- Bittner MI, Grosu AL, Brunner TB. Comparison of toxicity after IMRT and 3D-conformal radiotherapy for patients with pancreatic cancer - a systematic review. Radiother Oncol 2015;114:117-21. [Crossref] [PubMed]
- Hong TS, Ryan DP, Borger DR, et al. A phase 1/2 and biomarker study of preoperative short course chemoradiation with proton beam therapy and capecitabine followed by early surgery for resectable pancreatic ductal adenocarcinoma. Int J Radiat Oncol Biol Phys 2014;89:830-8. [Crossref] [PubMed]
- Nichols RC Jr, George TJ, Zaiden RA Jr, et al. Proton therapy with concomitant capecitabine for pancreatic and ampullary cancers is associated with a low incidence of gastrointestinal toxicity. Acta Oncol 2013;52:498-505. [Crossref] [PubMed]
- Sachsman S, Nichols RC, Morris CG, et al. Proton Therapy and Concomitant Capecitabine for Non-Metastatic Unresectable Pancreatic Adenocarcinoma. Int J Part Ther 2014;1:692-701. [Crossref]
- Terashima K, Demizu Y, Hashimoto N, et al. A phase I/II study of gemcitabine-concurrent proton radiotherapy for locally advanced pancreatic cancer without distant metastasis. Radiother Oncol 2012;103:25-31. [Crossref] [PubMed]
- Ito H, Kimura F, Shimizu H, et al. Surgical resection for pancreatic cancer combined with preoperative carbon-ion beam irradiation. Gan To Kagaku Ryoho 2004;31:1879-81. [PubMed]
- Shinoto M, Yamada S, Terashima K, et al. Carbon Ion Radiation Therapy With Concurrent Gemcitabine for Patients With Locally Advanced Pancreatic Cancer. Int J Radiat Oncol Biol Phys 2016;95:498-504. [Crossref] [PubMed]
- Okada T, Kamada T, Tsuji H, et al. Carbon ion radiotherapy: clinical experiences at National Institute of Radiological Science (NIRS). J Radiat Res 2010;51:355-64. [Crossref] [PubMed]
- Mendenhall NP, Malyapa RS, Su Z, et al. Proton therapy for head and neck cancer: rationale, potential indications, practical considerations, and current clinical evidence. Acta Oncol 2011;50:763-71. [Crossref] [PubMed]
- Thompson RF, Mayekar SU, Zhai H, et al. A dosimetric comparison of proton and photon therapy in unresectable cancers of the head of pancreas. Med Phys 2014;41:081711 [Crossref] [PubMed]
- Shinoto M, Ebner DK, Yamada S. Particle Radiation Therapy for Gastrointestinal Cancers. Curr Oncol Rep 2016;18:17. [Crossref] [PubMed]
Cite this article as: Ng SP, Koay EJ. Current and emerging radiotherapy strategies for pancreatic adenocarcinoma: stereotactic, intensity modulated and particle radiotherapy. Ann Pancreat Cancer 2018;1:22.