Immune defects in pancreatic cancer
Pancreatic cancer poorly responds to immunotherapy. In the past, immunotherapy focused on inducing tumor specific effector T cells (1). Adoptive T cell transfer and cancer vaccine have been two major approaches. However, there was little success, particularly in treating pancreatic cancer. For a long time, pancreatic cancer was not considered to be immunogenic. Therefore, treating the pancreatic cancer with cancer vaccine or adoptive T cell transfer was investigated in the clinical trials. Nevertheless, cancer vaccines carrying immune dominant antigens is still not effective even though vaccines were able to induce effector T cell responses in the peripheral blood (2). Not surprisingly, adoptive T cell transfer was also not successful. Then, it has been recognized that there must be some “defects” with pancreatic cancer, particularly in the tumor. Now, accumulated evidence has indicated that there are “immune defects” in the tumor microenvironment (TME) of pancreatic cancer.
Lack of “high-quality” T cells
The first immune defect in the pancreatic cancer is the lack of the effector T cells in the TME (3,4). Although there are still CD8+ T cells in the TME of pancreatic cancer, in the majority of pancreatic cancer, the numbers of CD8+ T cells are significantly smaller than those seen in the more immune active tumors or so-called “hot” tumors such as melanoma and renal cell carcinoma (5). There are many potential reasons. However, in the tumors of patients who received a cancer vaccine prior to the surgical resection of pancreatic cancer, intratumoral tertiary lymphoid aggregates were evidently induced, suggesting that, at least in the majority of pancreatic cancer patients, if T cells can be induced peripherally, they can enter the tumors (5). Nevertheless, intratumoral infiltration of T cells does not appear to result in an improved clinical outcome in these patients. On another hand, in a handful patients who have had a long-term survival following the surgical resection of their pancreatic cancer, high numbers of granzyme B+ T cells were observed in the TME of their tumors (6). Interestingly, their tumors also carry missense mutations that were predicted to produce cross-reactive microbial epitopes whereas patients with poor survival could have equal numbers of neoantigens predicted from the mutations in their tumors. Neoantigens that mimic microbial epitopes would be anticipated to less likely evade the immune rejection. These observations raised the hypothesis that a small subgroup of pancreatic cancer patients may be protected by “high-quality” antigen-specific T cells. Mismatch repair deficiency can also result in a high mutation burden in pancreatic cancer as seen in microsatellite instability (MSI)-high colorectal cancer and would lead to response to the immune checkpoint inhibitor (7). MSI-high pancreatic cancer is indeed infiltrated with a high number of CD8+ cells. Nevertheless, none of MSI-low or microsatellite stable pancreatic cancers were found to have a mutation burden comparative to MSI-high tumors; or rarely a pancreatic tumor carries a mutation burden at the level of that seen in melanoma or non-small cell lung cancer. Another possibility is that a dynamic mutagenesis conferred by MMR deficiency would increase the chance of generating “high quality” neoantigens. Therefore, one of the major “immune defects” of pancreatic cancer is the lack of effector T cells due to either low tumor mutation burden or lack of “high quality” neoantigens. Thus, to overcome this immune defect, a cancer vaccine approach or a T cell therapy would still be needed to prime the TME with T cells. Nevertheless, traditional approaches that target tumor-associated antigens may not be sufficient to overcome this defect; instead, targeting neoantigens may be necessary.
Formidable stroma
The second immune defect of pancreatic cancer is the barrier for the effector immune cells to get access to tumor cells (8,9). Although tertiary lymphoid aggregates were observed in the tumors from patients who received the cancer vaccine treatment, granzyme B+ cells were sparsely present in the vicinity of tumor cells even though higher infiltration of granzyme B+ CD8+ cells were shown to correlate with better survival (5). In addition, the CD8+ cells in the lymphoid aggregates still appeared to be kept distant from the tumor cells. Pancreatic cancer has a dense stroma which results in a high hydrostatic pressure in intratumoral vessels and limits the trafficking of lymphocytes. Therefore, it would be anticipated that targeting the dense stroma may facilitate the trafficking of lymphocytes (10,11). PEGPH20, a pegylated hyaluronidase that degrades hyaluronic acid, an extracellular matrix component in the dense stroma of pancreatic cancer is now being tested in a number of clinical concepts to examine its effect in overcoming the barrier for the effector immune cell infiltration in pancreatic cancer (12). In addition, immune cell trafficking is regulated by chemokines and their receptors. There are very few studies that can help us understand how to enhance the immune cell trafficking by targeting chemokines and chemokine receptors.
Immunosuppressive TME
The third immune defect of pancreatic cancer is the immunosuppressive TME in pancreatic cancer. Essentially in the TME of every pancreatic cancer, myeloid cells are a dominant immune cell type (13). How these myeloid cells become dominant in the TME of pancreatic cancer is not completely clear. However, one of the most popular hypotheses is that the genetic alternation in the pre-cancerous lesions, e.g., pancreatic intraepithelial neoplasia (PanIN), has induced a proinflammatory reaction. This proinflammatory reaction is not able to reject the pre-cancerous lesions that harbor the genetic alteration. Instead, it results in an infiltration of innate immune cells including macrophages and neutrophils (13). Then, the genetically altered neoplastic cells appear to have the capacity of reprogramming these myeloid cells from an anti-cancer condition into a pro-cancerous condition. These pro-cancerous myeloid cells including M2 macrophages and myeloid-derived suppressive cells (MDSC) are highly immunosuppressive (14). In the meantime, T cell differentiation also undergoes an abnormal process (15). The TME of pancreatic cancer is predominantly infiltrated with T regulatory cells. This third immune defect appears to be an important determinant of immune response after the tumors have been infiltrated with effector T cells. Essentially, all the tumors resected from patients who received the vaccine therapy are infiltrated abundantly with T cells; however, only those tumors infiltrated with fewer myeloid cells were associated with longer survival (16). Therefore, to overcome this third immune defect, one would need to target macrophages and other myeloid cells. However, simply depleting the innate immune cells may adversely deplete antigen-presenting cells. Thus, molecularly targeting myeloid cell function would be an ideal strategy. Our preclinical studies also suggest that activation receptors such as OX40 or CD137 are induced in T cells following the myeloid cell blockade treatment (17). This is not a surprise as the innate immune cells activate or suppress T cells through these activation receptors. Therefore, targeting the T cell activation process may also overcome the T cell suppression from the immunosuppressive TME in pancreatic cancer and warrants a further validation in the clinical testing.
Immune checkpoints
The fourth immune defect of pancreatic cancer is the immune checkpoint. However, different from “hot” tumors, pancreatic cancer does not respond to the immune checkpoint inhibitors. Thus, a defect in the immune checkpoint is often not considered. Indeed, an immune checkpoint mechanism is activated by many therapeutic modalities attempted for treating pancreatic cancer. For instant, vaccine therapy robustly induces PD-1+ T cells in the TME of pancreatic cancer (5). Presumably, the activation of immune checkpoints would immediately “disarm” the vaccine therapy. The activation of immune checkpoints is apparently a response to the induction of PD-L1 expression on the tumor epithelial cells and on the myeloid cells by vaccine therapy, likely through the interferon gamma signaling. Otherwise, in untreated pancreatic cancer, PD-L1 is scarcely expression. By contrast, PD-L1 expression can be evidently observed in treated pancreatic cancers including those treated by a cancer vaccine, chemotherapy, and/or radiation therapy (18). Certainly, the presence of immune checkpoint mechanisms does not mean that these treated pancreatic cancers would readily respond to immune checkpoint inhibitors. It should be recognized that induction of T cell activation and infiltration in pancreatic cancer is always accompanied by the activation of immune checkpoints. Therefore, blocking immune checkpoints is essential for treating pancreatic cancer, however, would not be sufficient as other immune defects are still present in pancreatic cancer.
Unfortunately, rarely a pancreatic cancer has no immune defect or has only one single defect. Most of the pancreatic cancer has more than two immune defects or all four immune defects (Figure 1). Individual tumors may have a different combination of the defects. Even though they may have the same type of immune defects among the four types of defects, the exact defect mechanisms could still be different. Therefore, to unlock the gate to a curative pathway for pancreatic cancer, a right sequential combination of therapeutic strategies that overcome multiple immune defects is the key.
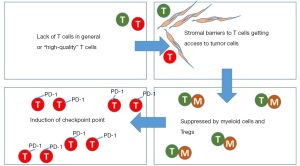
Acknowledgments
Funding: This work was supported in part by NIH grant R01 CA169702; NIH grant R01 CA197296; the Viragh Foundation and the Skip Viragh Pancreatic Cancer Center at Johns Hopkins; National Cancer Institute Specialized Programs of Research Excellence in Gastrointestinal Cancers grant P50 CA062924; Sidney Kimmel Comprehensive Cancer Center grant P30 CA006973.
Footnote
Provenance and Peer Review: This article was commissioned by the Guest Editors (Yingbin Liu and Wei Gong) for the series “The 8th Annual International Surgery Forum” published in Annals of Pancreatic Cancer. The article has undergone external peer review.
Conflicts of Interest: The author has completed the ICMJE uniform disclosure form (available at http://dx.doi.org/10.21037/apc.2018.11.01). The series “The 8th Annual International Surgery Forum” was commissioned by the editorial office without any funding or sponsorship. LZ serves as the unpaid Editor-in-Chief of Annals of Pancreatic Cancer. LZ reports grants from Merck, during the conduct of the study; grants from iTeos, grants from Bristol-Meyer Squibb, grants from Merck, grants from Astrazeneca, grants from Amgen, grants from NovaRock, grants from Inxmed, grants from Halozyme, personal fees from Biosion, personal fees and other from Alphamab, personal fees from NovaRock, personal fees from Akrevia/ Xilio, personal fees from Datarevive, personal fees from QED, personal fees from Natera, personal fees and other from Mingruizhiyao, outside the submitted work. The author has no other conflicts of interest to declare.
Ethical Statement: The author is accountable for all aspects of the work in ensuring that questions related to the accuracy or integrity of any part of the work are appropriately investigated and resolved.
Open Access Statement: This is an Open Access article distributed in accordance with the Creative Commons Attribution-NonCommercial-NoDerivs 4.0 International License (CC BY-NC-ND 4.0), which permits the non-commercial replication and distribution of the article with the strict proviso that no changes or edits are made and the original work is properly cited (including links to both the formal publication through the relevant DOI and the license). See: https://creativecommons.org/licenses/by-nc-nd/4.0/.
References
- Foley K, Kim V, Jaffee E, et al. Current progress in immunotherapy for pancreatic cancer. Cancer Lett 2016;381:244-51. [Crossref] [PubMed]
- Salman B, Zhou D, Jaffee EM, et al. Vaccine therapy for pancreatic cancer. Oncoimmunology 2013;2:e26662 [Crossref] [PubMed]
- Beatty GL, Chiorean EG, Fishman MP, et al. CD40 agonists alter tumor stroma and show efficacy against pancreatic carcinoma in mice and humans. Science 2011;331:1612-6. [Crossref] [PubMed]
- Clark CE, Hingorani SR, Mick R, et al. Dynamics of the immune reaction to pancreatic cancer from inception to invasion. Cancer Res 2007;67:9518-27. [Crossref] [PubMed]
- Lutz ER, Wu AA, Bigelow E, et al. Immunotherapy converts nonimmunogenic pancreatic tumors into immunogenic foci of immune regulation. Cancer Immunol Res 2014;2:616-31. [Crossref] [PubMed]
- Balachandran VP, Łuksza M, Zhao JN, et al. Identification of unique neoantigen qualities in long-term survivors of pancreatic cancer. Nature 2017;551:512-6. [PubMed]
- Le DT, Uram JN, Wang H, et al. PD-1 Blockade in Tumors with Mismatch-Repair Deficiency. N Engl J Med 2015;372:2509-20. [Crossref] [PubMed]
- Feig C, Gopinathan A, Neesse A, et al. The pancreas cancer microenvironment. Clin Cancer Res 2012;18:4266-76. [Crossref] [PubMed]
- Rucki AA, Zheng L. Pancreatic cancer stroma: understanding biology leads to new therapeutic strategies. World J Gastroenterol 2014;20:2237-46. [Crossref] [PubMed]
- Feig C, Jones JO, Kraman M, et al. Targeting CXCL12 from FAP-expressing carcinoma-associated fibroblasts synergizes with anti-PD-L1 immunotherapy in pancreatic cancer. Proc Natl Acad Sci U S A 2013;110:20212-7. [Crossref] [PubMed]
- Singha NC, Nekoroski T, Zhao C, et al. Tumor-associated hyaluronan limits efficacy of monoclonal antibody therapy. Mol Cancer Ther 2015;14:523-32. [Crossref] [PubMed]
- Hingorani SR, Zheng L, Bullock AJ, et al. HALO 202: Randomized Phase II Study of PEGPH20 Plus Nab-Paclitaxel/Gemcitabine Versus Nab-Paclitaxel/Gemcitabine in Patients With Untreated, Metastatic Pancreatic Ductal Adenocarcinoma. J Clin Oncol 2018;36:359-66. [Crossref] [PubMed]
- Zheng L, Xue J, Jaffee EM, et al. Role of immune cells and immune-based therapies in pancreatitis and pancreatic ductal adenocarcinoma. Gastroenterology 2013;144:1230-40. [Crossref] [PubMed]
- Greten TF. Myeloid-derived suppressor cells in pancreatic cancer: more than a hidden barrier for antitumour immunity? Gut 2014;63:1690-1. [Crossref] [PubMed]
- Seo YD, Pillarisetty VG. T-cell programming in pancreatic adenocarcinoma: a review. Cancer Gene Ther 2017;24:106-13. [Crossref] [PubMed]
- Tsujikawa T, Kumar S, Borkar RN, et al. Quantitative Multiplex Immunohistochemistry Reveals Myeloid-Inflamed Tumor-Immune Complexity Associated with Poor Prognosis. Cell Rep 2017;19:203-17. [Crossref] [PubMed]
- Saung MT, Muth S, Ding D, et al. Targeting myeloid-inflamed tumor with anti-CSF-1R antibody expands CD137+ effector T-cells in the murine model of pancreatic cancer. J Immunother Cancer 2018;6:118. [Crossref] [PubMed]
- Zheng L. PD-L1 Expression in Pancreatic Cancer. J Natl Cancer Inst 2017;109: [Crossref] [PubMed]
Cite this article as: Zheng L. Immune defects in pancreatic cancer. Ann Pancreat Cancer 2018;1:33.