Volatile organic compound analysis for the diagnosis of pancreatic cancer
Introduction
Cancer is a devastating disease that represents the second leading cause of death worldwide, responsible for an estimated 9.6 million deaths in 2018 (1). Pancreatic cancer is particularly lethal, representing the 5th deadliest cancer in the UK despite being only the 11th most common (2). This is reflected in its dismal 5-year survival rate of 9% (3). This poor survival is largely attributed to late diagnosis (4,5), with only 10–20% of individuals having surgical resectable disease at the time of its detection. With surgery currently the only potentially curative therapy, earlier diagnosis of pancreatic cancer is urgently needed in order to improve survival outcomes (3).
The World Health Organisation (WHO) has stated that there are two main components for early detection: education and population screening (1). Increased awareness of the early warning signs of cancer can have a significant impact in some cancer types. However sadly, in pancreatic cancer, distinct clinical symptoms typically only manifest in the advanced stages of the disease. Even when they develop, symptoms can be vague in nature and are often mistaken for other conditions, such as irritable bowel syndrome (3,4).
Abdominal ultrasound is a non-invasive low-cost modality that is often used as first line investigation for patients presenting with symptoms. However, its sensitivity is user-dependent and it often struggles to detect smaller tumours (6). Thus, complementary investigations are often performed to achieve a diagnosis [such as computed tomography (CT) scans, endoscopic retrograde cholangiopancreatography (ERCP) and endoscopic ultrasonography (EUS)]. These tests are expensive and can be unpleasant or pose significant clinical risks to the patient (e.g., radiation exposure or infection). These factors, alongside the low prevalence of pancreatic cancer within the general population (UK age-standardised incidence rate of 16.9 per 100,000), means that screening of the disease unfeasible under current circumstances (2).
With the incidence of pancreatic cancer rising, developing additional tools to facilitate screening in asymptomatic individuals is essential. A successful approach to a screening strategy might be to develop a cheap, effective and acceptable test which selects out those patients likely to benefit from more invasive, complex and expensive interventions. In light of this, a considerable amount of research has been completed over the last few decades to develop various diagnostic biomarkers. However, while many potential candidate biomarkers for the disease have been developed, pancreatic cancer continues to lack tests with sufficient applicability, acceptability, specificity, and sensitivity (7).
Analysis of volatile organic compounds (VOC) produced by pancreatic cancer cells and/or the tumour microenvironment, which are detectable either in the headspace of or within different bodily fluids is a promising technique for the development of novel biomarkers. VOCs generated through cellular metabolism are released into the circularity system before being excreted from the body. As such, examination of the VOC profile can give a holistic snapshot of the metabolic state of an organism, reflecting biological activities such as cell death, inflammation and oxidative stress (8). Cancer-related alterations in cell metabolism result in marked shifts of the spectra and concentrations of the VOCs produced, both systemically and locally (8). The patterns of change are unique and specific to each disease and as such the last few decades have seen extensive efforts to develop VOC biomarkers, by identifying key differences between healthy controls and individuals with cancer.
This review critically examines the progress made in the development of VOC analysis, as a diagnostic tool in early pancreatic cancer. We also examine the challenges and limitations of this field in both the present and the future.
Current evidence regarding the analysis of VOCs in pancreatic cancer
In 1985, Gordon et al. (9) published a landmark study demonstrating the ability of VOCs, found in exhaled breath, to aid in the diagnosis of lung cancer. As a result of this, interest into the clinical diagnostic potential of VOCs within cancer care has significantly risen within the last 30 years. An electronic literature search was performed on the PubMed and Embase databases during May 2020. The key words (cancer OR carcinoma OR adenocarcinoma OR tumor OR tumour OR malignancy OR malignant disease) AND (Pancreatic OR Pancreas OR PDAC OR Pancreatic ductal adenocarcinoma) AND ((volatile AND (compound OR compounds OR marker OR markers OR biomarker OR biomarkers)) OR VOC OR VOCs) were used. This search identified 30 potential papers. One reviewer (B.T.C.) assessed the abstracts for eligibility revealing 7 suitable publications (Table 1) which included data from 10 different cancer sites. Papers were excluded if they did not include data relevant to pancreatic cancer, were not specific to VOCs or did not include human participants. A flow diagram depicting the process of selecting papers can be found in Figure 1.
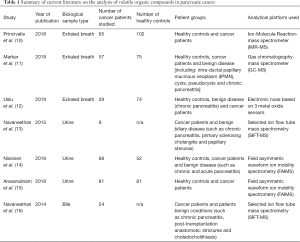
Full table
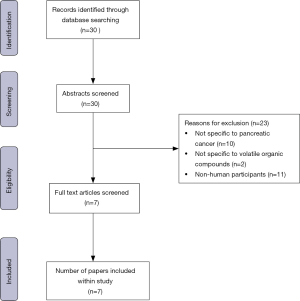
The majority of publications highlighted in this review represent phase 1 biomarker discovery studies, comparing patients with cancer to a healthy control population and/or those with benign diseases of the pancreas (e.g., chronic pancreatitis). Several different strategies were utilised to differentiate between cancer and control cases. Some studies developed models for diagnosis based on specific concentrations, whereas other groups assessed the general spectral pattern using numerous data points (Table 2). The variation between analytical techniques and biological sample types makes the comparison of data between studies difficult. However, where specifically identified the majority of biomarkers were alkanes, alkenes, alcohols, aldehydes or ketones. The compounds produced are not in themselves specific to pancreatic cancer, being shown to be produced in a variety of cancers (8,17,18); it is rather the unique VOC production pattern that is unique to the disease. A combination of general cancer biomarkers and specific biomarkers would be very promising for the detection of pancreatic cancer. However, it is important to note that current studies vary significantly in sampling procedure, size/type of patient groups and the analytical platform used. Standardization of methodology is crucial if VOCs analysis is to be utilised clinically. Though despite this, the diagnostic potential of VOCs profile analysis in the early detection of pancreatic cancer is still promising.
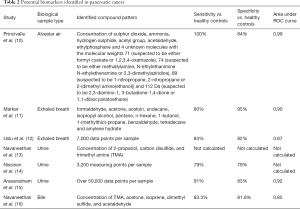
Full table
All published papers used area under the curve (AUC) to assess the inherent validity of each diagnostic test. The AUC is an effective and combined measure of sensitivity and specificity that describes the effectiveness of diagnostic tests (19). Despite is merits, calculating AUC and summarizing data over all possible thresholds can be misleading and prone to potential bias (20). As ROC curves and AUC are threshold-independent, they describe a classifier’s performance over the entire operating range, including regions of no practical clinical relevance such as the extremes of a high false-positive or low true-positive rate.
Biological sample types
Three biological sample types (exhaled breath, urine and bile) have been analysed for use in the diagnosis of pancreatic cancer. Analysis of exhaled breath has the potential to be clinically useful. Being non-invasive in nature it allows samples to be collected at the point of care in varying quantities. In addition, exhaled breath does not require any special storage conditions as other samples do (11). Three papers explored breath analysis of VOCs in pancreatic cancer identifying a significant number of biomarkers present (10-12). Analysis of VOCs in exhaled breath demonstrated a significant ability to distinguish between cancer patients and healthy controls with a mean area under the ROC curve of 0.92, a pooled sensitivity of 87.67%, and a pooled specificity of 87.0%. Despite great promise, compounds in breath analysis are often exogenous in nature, i.e., they have not been generated by the cancer. Phillips et al. (21) estimate that only half of the 3,500 VOCs in exhaled breath are endogenous leading to doubts about the reliability of the results.
VOCs detected in internal biological matrices such as urine and bile can also be exogenous in origin. Once inhaled, exogenous VOCs may dissolve into the bloodstream and subsequently be excreted in bodily fluids. Urine samples are also non-invasive in nature but have the advantage over exhaled breath in that analytes are often concentrated by the kidneys prior to excretion (22). However, urine is particularly affected by drugs administered to the patient. Considering this, the VOC profile impact of specific therapies must be taken into account when analysing urine samples. Three papers have been published investigating the use of VOCs in urine for pancreatic cancer (13-15), identifying three alternative models. Analysis of VOCs in urine also demonstrated a significant ability to distinguish between cancer patients and healthy controls with a mean area under the ROC curve of 0.92, a pooled sensitivity of 85.0%, and a pooled specificity of 81.0%.
Navaneethan et al. (16) identified bile collected during ERCP as a potential sample type. They identified 5 potential biomarkers, with trimethyl amine (TMA) also being found in urine. These biomarkers helped develop a diagnostic model with an area under the ROC curve of 0.85, a sensitivity of 83.3%, and a specificity of 81.9%. The direct aspiration of bile is an invasive procedure and thus there are inherent risks to the patient. However, if utilised within current ERCP testing, there is potential to increase both sensitivity and specificity of diagnosis with minimal additional risks to the patient.
Mechanism of production
Another potential challenge of VOC analysis is that despite substantial research the exact mechanism and origin of VOCs remains relatively unknown, with no guarantee that detected compounds are produced or consumed by cancer cells. VOC patterns also differ naturally between individuals due to genetic differences, environmental influences, diet, and smoking (8). Nissinen et al. (14) has speculated however that the specific VOC production pattern in pancreatic cancer is perhaps related to an inflammatory response within the pancreas. They suggested that this hypothesis is supported by the inability of VOCs to distinguish between cancer and pancreatitis (both acute and chronic). Despite the exact mechanism being unclear, VOC profiles appear to be specific to pancreatic pathology, indicating significant differences as compared to pathologies of other organs (8,17,18), and distinct patterns being observed in the bile fluid (16). Further research into the pathophysiology underlying the alteration in the spectra and origin of VOCs is essential to enable refinement of VOC technology.
Volatolome analytical platforms
Concentrations of most of the VOCs present in biological matrices are low: in the nmol−1 − pmol−1 range in exhaled human breath and bile, and in the µmol−1 to nmol−1 range in urine and blood (23-25). In addition, VOCs often form part of complex mixtures. Therefore, the quantification of VOCs presents significant challenges. Whilst many analytical techniques exist for the analysis of VOCs, the majority of publications related to pancreatic cancer (1,6) utilise mass-spectrometry based chemical analysis technology. This can be used to detect specific VOCs and their abundances, via the identification of specific properties in each component of a sample. Despite relying on the same underlying technology, it is important to note that significant variation in methodology exists between the different subtypes of mass spectrometry. Each subtype has their uses, advantages and disadvantages.
Providing both qualitative and quantitative information, gas-chromatography mass spectrometry (GC-MS) is often considered the gold standard technique for VOC analysis, allowing access to the full VOC composition of a sample (26). In this process, a micro-extraction fibre is exposed to the headspace above the sample for a set period of time, this allows VOCs to adsorb into the fibre, pre-concentrating the sample before analysis.
This initial phase is called solid-phase micro-extraction (SPME). The VOCs are then thermally desorbed (220C) from the micro-extraction fibre and introduced to a gas chromatograph (GC) with high purity helium (the mobile phase). Due to the variation in elution times, this separates the sample into its constituent VOCs in separating columns. Following elution of individual VOCs, a downstream mass spectrometer (MS) ionises the VOCs into fragments. This allows for the identification of chemical structures by deriving the mass/charge (m/z) ratios of each individual VOC’s constituent fragments (26-29). A schematic summary of this process can be found in Figure 2. GC-MS is a powerful analytical technique regularly used within the identification, detection and quantification of compounds. However, due to its relatively high limit of detection, its application can sometimes be difficult, sample pre-concentration and daily calibration, by expert technicians, is often required. This pre-concentration step is the most labour-intensive part of the process, as well as the primary source of errors within the analysis, ultimately influencing the reliability and accuracy of the system (30). As such, its high operating costs render it prohibitively expensive for use as a clinical diagnostic tool, explaining why it was only used in a single paper.
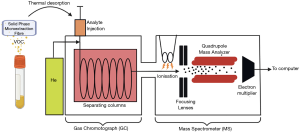
Three of the studies (10,13,16) utilised direct injection mass-spectrometry methods; two used selected ion flow tube mass spectrometry (SIFT-MS) and one used ion-molecule reaction mass spectrometry (IMR-MS). The electron impact ionisation, of conventional mass-spectrometers, causes high fragmentation of molecules in a complex gas mixture, often producing highly complex mass spectra, with overlapping intensities (31). This makes quantification difficult or impossible. In IMR-MS and SIFT-MS, there is little or no pre-separation of the sample, leading to reduced fragmentation and enhanced quantification (25). They are also faster than GC-MS and provide real-time absolute concentration of several VOCs simultaneously. Furthermore, these direct methods have lower limits of detection than traditional GC-MS technology (32,33). However, they also are less selective and are unable to quantify unknown compounds (34,35). In this way, GC-MS could be used to complement the fast screening capabilities of direct injection techniques, with a slower but more selective analysis.
Finally, two of the studies (14,15) utilised field asymmetric ion mobility spectrometry (FAIMS), a method used to distinguish between charged gaseous molecules based upon their behaviour in both weak and strong electrical fields (36). In this technique, a sample is ionised and then the ions are passed through a high electric field, created by applying an asymmetric high voltage to parallel magnetic plates. The electric charge and mass of these ions will determine the degrees of movement, towards and away from the electrode, producing the waveform as ions transit the channel between the plates towards an ion sensor. Two variables are altered: the magnitude of the electric field, or ‘dispersion field’ measured between 0% and 90%, and the ‘compensation voltage’ measured between −6V and +6V, which is used to remove the drift of ions produced by the high electric field. As each of these variables are altered over the course of sample analysis, only molecules with specific mobilities can exit the electrode tunnel at specific values of dispersion field and compensation voltage (Figure 3) (37). Compensation voltage is then plotted against dispersion field, and the values of both, when ions reach the sensor at the end of the tube, are plotted as a heatmap based on the abundance of ions (or ‘ion current’) reaching the ion detector at those specific values. These produce ‘plumes’, which can be compared between different sample groups using Fisher discrimination analysis to determine group-level differences (38). This technique has the advantage of being performed at room temperature and pressure, without the need for preparation of the sample (36). Like IMR-MS and SIFT-MS, FAIMS is highly sensitive (24). However, one limitation of this technique is the difficulty reproducing results on different instruments. Two different instruments may separate ions at slightly different voltages making the comparison of data between groups difficult (39,40).
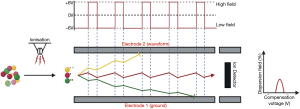
In addition to mass-spectrometry based technology, one study (12) used an electronic nose, a device which mimics the human olfactory system. A variety of these devices have been developed but they generally consist of a micro-array of sensors, which differ in polarity from one another [3 metal oxide sensors in the case of Uslu et al. (12)]. The sample gas passes through these arrays, absorbing to various degrees depending on chemical composition. The level of compound absorption on the sensors alters the mass of resistance; it is this change that is then detected by a computer. The computer recognises the overall pattern of the gas, detecting differences between samples using programmes, rather than quantifying individual VOCs. This is often considered to be a limitation of the technique as the concentration of individual biomarkers is unable to be obtained (25). Before electronic noses can be used routinely within the diagnosis of cancer, it is first necessary to identify and refine the characteristic pattern of VOCs seen in cancer patients. There is however great promise for electronic noses, due to their ability to provide rapid results and their relative ease of use at point of care (41).
More refinement, as well as standardisation of analytical and sampling technologies, is still required if VOC analysis is to be developed for diagnostics. Although it is more likely that any future device will be an electronic nose, due to its cheaper cost, practicality of use and speed of results, all the analytical techniques have their individual uses, advantages and disadvantages. A summary of the main techniques is shown within Table 3.
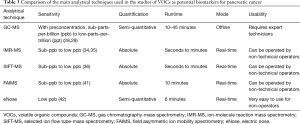
Full table
Several free-to access databases have been constructed to aid in the analysis of large complex datasets of VOC analysis (42,43). Due to the relatively few publications assessing specific pancreatic VOC’s however, their current applicability is somewhat limited.
The future
In terms of diagnostic validity, due to the inconsistency between study designs, it would perhaps be premature to declare any excreta superior to another for identifying pancreatic cancer. Despite this, preliminary results appear to establish the great promise of VOC analysis as a diagnostic tool.
A pooled analysis of all the current literature related to pancreatic cancer, highlights the significant potential of the technique: sensitivities of 87.67%, 85.00% and 83.30% as well as specificities of 87.00%, 81.00% and 81.90% have all been shown for breath, urine and bile respectively. Whilst VOC analysis currently has a lower sensitivity as compared to EUS and ERCP (Table 4), its non-invasive, inexpensive nature certainly makes it an attractive alternative. However, both the sensitivity and specificity, is notably greater in all biological matrices compared to Ca-19-9, the biomarker currently used for pancreatic cancer diagnosis. Further refinement and validation of, both normal and diseased, VOC profiles through large scale, multicentre clinical trials are however essential prior to clinical implementation.
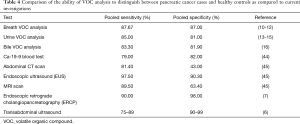
Full table
VOC analysis could be implemented at a variety of locations within the cancer care pathway (Figure 4). First, it could be utilised to triage patients presenting with non-specific symptoms, indicating to clinicians which patients require more invasive investigations, e.g., CT scans. Prior to implementation however, further study is undoubtably needed. One potential method of further refinement would be to further compare non-invasive VOC profiles in those patients already deemed as high-risk under current triaging processes (for example such as those undergoing invasive procedures for suspected pathology) to healthy individuals, who are currently deemed as low risk.
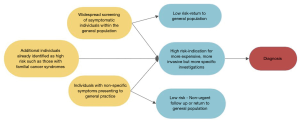
Secondly, whilst not discussed within this review, VOC analysis may have a role in monitoring an individual’s response to therapy. Being non-invasive and easy to perform there is potential for tests to also be developed for screening disease recurrence. Before this is done however, more research needs to occur to investigate the scope of VOCs abilities and whether this is applicable within pancreatic cancer. Third, if developed to the point of acceptable sensitivity and selectivity, VOC analysis may have a role within screening of the general population. However, implementation of VOC analysis for a screening programme in pancreatic cancer requires careful consideration. If it were to be utilised there are two types of proposed designs: either a central/regional laboratory model or a point-of-care model. In a laboratory-based approach samples would be collected within the clinical environment before then being sent to a laboratory for analysis. This method presents significant challenges within the design of collection devices, as well as in the methodology of sample storage. However, it does allow for the application of various quality assurance methods, ensuring robust and reliable results (41). The second model is the development of a point-of-care device. This method has significant advantages in that it allows clinicians to make immediate decisions based on the results. However, as stated, further research and development, aimed at increasing the sensitivity and selectivity, is required prior to integration within a real clinical environment. As such other analytical methods should be used to compliment further research.
Conclusions
Early detection has the potential to increase the survival rate of pancreatic cancer significantly (1). Analysis of VOCs is an attractive option to achieve this, being relatively cheap, easy to use and non-invasive in nature as compared to other investigations. However, for VOC analysis to be developed the following challenges must be met: (I) knowledge of the origin of VOCs; (II) analytical techniques must be developed, refined and standardised to allow for reproducibility; (III) VOC models of healthy individuals and cancer cases must be refined to allow for accuracy to be developed; (IV) techniques must be validated through large-scale, multicentre clinical trials. However, despite these challenges, the diagnostic potential of VOCs in the early detection of pancreatic cancer is promising.
Acknowledgments
Funding: None.
Footnote
Conflicts of Interest: All authors have completed the ICMJE uniform disclosure form (available at http://dx.doi.org/10.21037/apc-20-39). The authors have no conflicts of interest to declare.
Ethical Statement: The authors are accountable for all aspects of the work in ensuring that questions related to the accuracy or integrity of any part of the work are appropriately investigated and resolved. All figures and tables produced as part of this paper are original and created by the named authors.
Open Access Statement: This is an Open Access article distributed in accordance with the Creative Commons Attribution-NonCommercial-NoDerivs 4.0 International License (CC BY-NC-ND 4.0), which permits the non-commercial replication and distribution of the article with the strict proviso that no changes or edits are made and the original work is properly cited (including links to both the formal publication through the relevant DOI and the license). See: https://creativecommons.org/licenses/by-nc-nd/4.0/.
References
- Organisation WH. Cancer. 2018. Available online: https://www.who.int/news-room/fact-sheets/detail/cancer
- UK PC. Pancreatic cancer statistics. 2018. Available online: https://www.pancreaticcancer.org.uk/what-we-do/media-centre/pancreatic-cancer-statistics/
- Rawla P, Sunkara T, Gaduputi V. Epidemiology of Pancreatic Cancer: Global Trends, Etiology and Risk Factors. World J Oncol 2019;10:10-27. [Crossref] [PubMed]
- Kommalapati A, Tella SH, Goyal G, et al. Contemporary Management of Localized Resectable Pancreatic Cancer. Cancers (Basel) 2018;10:24. [Crossref] [PubMed]
- Javed AA, Wright MJ, Siddique A, et al. Outcome of Patients with Borderline Resectable Pancreatic Cancer in the Contemporary Era of Neoadjuvant Chemotherapy. J Gastrointest Surg 2019;23:112-21. [Crossref] [PubMed]
- Ashida R, Tanaka S, Yamanaka H, et al. The Role of Transabdominal Ultrasound in the Diagnosis of Early Stage Pancreatic Cancer: Review and Single-Center Experience. Diagnostics (Basel) 2018;9:2. [Crossref] [PubMed]
- Poruk KE, Firpo MA, Adler DG, et al. Screening for pancreatic cancer: why, how, and who? Ann Surg 2013;257:17-26. [Crossref] [PubMed]
- Hakim M, Broza YY, Barash O, et al. Volatile organic compounds of lung cancer and possible biochemical pathways. Chem Rev 2012;112:5949-66. [Crossref] [PubMed]
- Gordon SM, Szidon JP, Krotoszynski BK, et al. Volatile organic compounds in exhaled air from patients with lung cancer. Clin Chem 1985;31:1278-82. [Crossref] [PubMed]
- Princivalle A, Monasta L, Butturini G, et al. Pancreatic ductal adenocarcinoma can be detected by analysis of volatile organic compounds (VOCs) in alveolar air. BMC Cancer 2018;18:529. [Crossref] [PubMed]
- Markar SR, Brodie B, Chin ST, et al. Profile of exhaled-breath volatile organic compounds to diagnose pancreatic cancer. Br J Surg 2018;105:1493-500. [Crossref] [PubMed]
- Uslu HI, Dölle AR, Dullemen HM, et al. Pancreatic ductal adenocarcinoma and chronic pancreatitis may be diagnosed by exhaled-breath profiles: a multicenter pilot study. Clin Exp Gastroenterol 2019;12:385-90. [Crossref] [PubMed]
- Navaneethan U, Parsi MA, Lourdusamy D, et al. Volatile Organic Compounds in Urine for Noninvasive Diagnosis of Malignant Biliary Strictures: A Pilot Study. Dig Dis Sci 2015;60:2150-7. [Crossref] [PubMed]
- Nissinen SI, Roine A, Hokkinen L, et al. Detection of Pancreatic Cancer by Urine Volatile Organic Compound Analysis. Anticancer Res 2019;39:73-9. [Crossref] [PubMed]
- Arasaradnam RP, Wicaksono A, O'Brien H, et al. Noninvasive Diagnosis of Pancreatic Cancer Through Detection of Volatile Organic Compounds in Urine. Gastroenterology 2018;154:485-7.e1. [Crossref] [PubMed]
- Navaneethan U, Parsi MA, Gutierrez NG, et al. Volatile organic compounds in bile can diagnose malignant biliary strictures in the setting of pancreatic cancer: a preliminary observation. Gastrointest Endosc 2014;80:1038-45. [Crossref] [PubMed]
- Qin T, Liu H, Song Q, et al. The screening of volatile markers for hepatocellular carcinoma. Cancer Epidemiol Biomarkers Prev 2010;19:2247-53. [Crossref] [PubMed]
- Peng G, Hakim M, Broza YY, et al. Detection of lung, breast, colorectal, and prostate cancers from exhaled breath using a single array of nanosensors. Br J Cancer 2010;103:542-51. [Crossref] [PubMed]
- Kumar R, Indrayan A. Receiver operating characteristic (ROC) curve for medical researchers. Indian Pediatr 2011;48:277-87. [Crossref] [PubMed]
- Adams NM, Hand DJ. Comparing classifiers when the misallocation costs are uncertain. Pattern Recognition 1999;32:1139-47. [Crossref]
- Phillips M, Gleeson K, Hughes JM, et al. Volatile organic compounds in breath as markers of lung cancer: a cross-sectional study. Lancet 1999;353:1930-3. [Crossref] [PubMed]
- Rondanelli M, Perdoni F, Infantino V, et al. Volatile Organic Compounds as Biomarkers of Gastrointestinal Diseases and Nutritional Status. J Anal Methods Chem 2019;2019:7247802 [Crossref] [PubMed]
- Deng C, Zhang X. A simple, rapid and sensitive method for determination of aldehydes in human blood by gas chromatography/mass spectrometry and solid-phase microextraction with on-fiber derivatization. Rapid Commun Mass Spectrom 2004;18:1715-20. [Crossref] [PubMed]
- Phillips M. Method for the collection and assay of volatile organic compounds in breath. Anal Biochem 1997;247:272-8. [Crossref] [PubMed]
- Manolis A. The diagnostic potential of breath analysis. Clin Chem 1983;29:5-15. [Crossref] [PubMed]
- Ahn JW, Pandey SK, Kim KH. Comparison of GC-MS Calibration Properties of Volatile Organic Compounds and Relative Quantification Without Calibration Standards. J Chromatogr Sci 2011;49:19-28. [Crossref]
- Schmidt K, Podmore I. Current Challenges in Volatile Organic Compounds Analysis as Potential Biomarkers of Cancer. J Biomark 2015;2015:981458 [Crossref] [PubMed]
- Fuchs P, Loeseken C, Schubert JK, et al. Breath gas aldehydes as biomarkers of lung cancer. Int J Cancer 2010;126:2663-70. [PubMed]
- Ligor T, Ligor M, Amann A, et al. The analysis of healthy volunteers' exhaled breath by the use of solid-phase microextraction and GC-MS. J Breath Res 2008;2:046006 [Crossref] [PubMed]
- Rosenthal D. Theoretical Limitations of Gas Chromatographic/Mass Spectrometric Identification of Multicomponent Mixtures. Analytical Chemistry 1982;54:63-6. [Crossref]
- Kivirand K. Biosensing Technologies for the Detection of Pathogens - A Prospective Way for Rapid Analysis. IntechOpen; 2018.
- Cauchi M, Fowler DP, Walton C, et al. Comparison of GC-MS, HPLC-MS and SIFT-MS in conjunction with multivariate classification for the diagnosis of Crohn's disease in urine. Analytical Methods 2015;7:8379-85. [Crossref]
- Tegtmeyer U, Weiss HP, Schlögl R. Gas analysis by IMR-MS: a comparison to conventional mass spectrometry. Fresenius' Journal of Analytical Chemistry 1993;347:263-8. [Crossref]
- Dolch ME, Frey L, Hornuss C, et al. Molecular breath-gas analysis by online mass spectrometry in mechanically ventilated patients: a new software-based method of CO(2)-controlled alveolar gas monitoring. J Breath Res 2008;2:037010 [Crossref] [PubMed]
- Smith D, Spanel P. Selected ion flow tube mass spectrometry (SIFT-MS) for on-line trace gas analysis. Mass Spectrom Rev 2005;24:661-700. [Crossref] [PubMed]
- Zrodnikov Y, Davis CE. The Highs and Lows of FAIMS: Predictions and Future Trends for High Field Asymmetric Waveform Ion Mobility Spectrometry. J Nanomed Nanotechnol 2012;3:109e. [Crossref] [PubMed]
- Swearingen KE, Moritz RL. High-field asymmetric waveform ion mobility spectrometry for mass spectrometry-based proteomics. Expert Rev Proteomics 2012;9:505-17. [Crossref] [PubMed]
- Griffiths RL, Creese AJ, Race AM, et al. LESA FAIMS Mass Spectrometry for the Spatial Profiling of Proteins from Tissue. Anal Chem 2016;88:6758-66. [Crossref] [PubMed]
- Chavarria MA, Matheoud AV, Marmillod P, et al. High sensitivity field asymmetric ion mobility spectrometer. Rev Sci Instrum 2017;88:035115 [Crossref] [PubMed]
- Oh EH, Song HS, Park TH. Recent advances in electronic and bioelectronic noses and their biomedical applications. Enzyme Microb Technol 2011;48:427-37. [Crossref] [PubMed]
- Behera B, Joshi R, Anil Vishnu GK, et al. Electronic nose: a non-invasive technology for breath analysis of diabetes and lung cancer patients. J Breath Res 2019;13:024001 [Crossref] [PubMed]
- Janfaza S, Banan Nojavani M, Khorsand B, et al. Cancer Odor Database (COD): a critical databank for cancer diagnosis research. Database (Oxford) 2017;2017.
- Agarwal SM, Sharma M, Fatima S. VOCC: a database of volatile organic compounds in cancer. RSC Advances 2016;6:114783-9. [Crossref]
- Ballehaninna UK, Chamberlain RS. The clinical utility of serum CA 19-9 in the diagnosis, prognosis and management of pancreatic adenocarcinoma: An evidence based appraisal. J Gastrointest Oncol 2012;3:105-19. [PubMed]
- Costache MI, Costache CA, Dumitrescu CI, et al. Which is the Best Imaging Method in Pancreatic Adenocarcinoma Diagnosis and Staging - CT, MRI or EUS? Curr Health Sci J 2017;43:132-6. [PubMed]
Cite this article as: Crosby BT, Ridzuan-Allen A, O’Neill JP. Volatile organic compound analysis for the diagnosis of pancreatic cancer. Ann Pancreat Cancer 2021;4:4.