The anti-metastasis effect of low-dose carbon monoxide
Most cancer deaths are due to metastasis and its negative impacts on the function of vital organs. At the time of primary cancer diagnosis, tumor cells in many patients have already spread to distant organs and will become metastatic disease if these microscopic foci of disseminated tumor cells (microscopic metastases) are left untreated. Once diagnosed with metastatic disease, it is notoriously difficult to treat. Pancreatic cancer is projected to become the second leading cause of cancer-related death by 2030. About 48% of pancreatic cancer patients are diagnosed with distant metastases (1), with the liver being the most common site of metastasis. Pancreatic cancer can be divided into exocrine tumors and pancreatic neuroendocrine tumors (PNET). The majority of pancreatic cancer is pancreatic ductal adenocarcinoma (PDAC), one type of exocrine tumors. PDAC is among the most aggressive of all cancers. Potentially curative surgical resection is limited to those PDAC patients diagnosed at an early stage, yet many of them ultimately experience recurrence (2). For those with metastatic PDAC, standard chemotherapy only marginally extends survival. Overall, the 5-year survival rate of PDAC is ~11% (1), underscoring the urgent need for novel therapies.
Carbon monoxide (CO) is an important cell signaling molecule produced endogenously by heme degradation (3). Research has uncovered therapeutic use for CO when applied at low concentrations for various non-cancerous diseases (4-6). Clinical trials of inhaled CO to treat pulmonary diseases demonstrated no adverse events after 12 weeks of inhaled low-dose CO therapy (7). Low-dose CO has therapeutic potential in inhibiting primary tumor growth in mouse models and sensitizing chemotherapeutic agents (8). Vítek et al. (9) reported that 500 ppm CO (1 hour daily) reduced the subcutaneous tumor burden of human PDAC cell line xenograft in athymic mice and increased the survival rate twofold compared with the control mice. However, the subcutaneous tumor mouse model cannot address the metastatic nature, important determinant of the therapeutic response.
In a recent study, Zhang et al. (10) provided the direct evidence for the effect of low-dose CO (250 ppm, 3 hours daily) in suppressing metastasis using two clinically relevant mouse models. First, the authors demonstrated that low-dose CO significantly inhibited liver metastasis of PDAC using an orthotopic mouse model of liver metastasis by intrasplenically injecting human PDAC cell lines (11). Treatment of 250 ppm CO (3 hours daily) significantly blocked the metastatic outgrowth of PDAC in the liver. Second, because of the high recurrence rates in early-stage PDAC patients despite surgical resection followed by chemotherapy (12), the authors investigated the use of low-dose CO as an adjuvant therapy to prevent the progression of microscopic metastasis to gross metastasis. To mimic the clinic setting, the authors developed a new spontaneous PDAC metastasis mouse model by growing human PDAC cells subcutaneously in NOD/scid-lL2Rgc knockout (NSG) immunodeficient mice and then resecting tumors when their sizes reached 0.4 cm in diameter. In this model, mice succumbed to recurrence with PDAC metastases in the liver and the peritoneal cavity 4 weeks post-surgery. Importantly, the authors found that low-dose CO treatment significantly suppressed recurrence after surgical removal of primary PDAC. In addition, the authors demonstrated the therapeutic effect of low-dose CO in blocking lung metastasis in a triple-negative breast cancer mouse model, which broadens the impact of low-dose CO therapy in treating other aggressive metastatic cancer types. Of an important note, mice treated with low-dose CO (250 ppm, 3 hours daily) in all three metastasis mouse models examined by Zhang et al. remained healthy when the untreated mice became lethargic and needed to be euthanized as metastatic diseases developed.
Mechanistically, Zhang et al. found that low-dose CO blocked the transcription of heme importers, HRG1 and HCP1, leading to diminished intracellular heme levels, a heme-regulated enzyme, cytochrome P4501B1 (CYP1B1), and its downstream molecules, including SP1, Myc, and Myc target genes (10). It has been reported that elevated heme levels are found in cancer cells and contribute to tumorigenesis (13,14); heme upregulates c-Myc expression in prostate cancer (14); siRNA against HRG1 impairs migration of HeLa cells (15); CYP1B1 promotes migration and invasion (16); CYP1B1 overexpression is associated with poor response to chemotherapy (17). These earlier studies indicate the roles of heme, Hrg1, and CYP1B1 in metastasis. Zhang et al. showed that either supplementing heme or overexpressing CYP1B1 reverses the anti-migration effect of low-dose CO in vitro. Because Myc regulates cancer metabolism (18), the authors performed polar metabolite profiling by liquid chromatography-mass spectrometry (LC-MS) and a pathway topology analysis through which they found that the TCA cycle was significantly downregulated in the CO-treated cells. On the contrary, hypoxia was not induced in the low-dose CO-treated tumor cells as measured by HIF1α and HIF2α protein levels. Whether low-dose CO therapy achieves its anti-metastatic effect by downregulating intracellular heme levels, the CYP1B1/Sp1/Myc axis, or the TCA cycle in vivo and in various cancer types requires further investigation (Figure 1). Heme is a regulatory molecule exerting functional consequences via transient binding to many proteins. Besides CYP1B1, other heme-regulated proteins may contribute to the anti-metastatic effect of low-dose CO. Taken together, this work by Zhang et al. provides strong evidence to repurpose low-dose CO for the inhibition of metastatic cancer development.
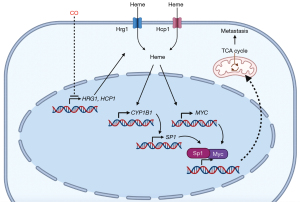
Looking into the future, we see great potential for developing CO as a therapeutic agent against metastasis. On the one hand, the property of CO makes it an excellent candidate for systemic therapy of metastatic cancer. CO is very stable and is known to be highly permeable and diffusible through the endothelium [review in (3)]. Additional investigation is needed to determine whether low-dose CO can lead to the regression of metastatic cancer. As the immune system is increasingly recognized to play a critical role in cancer progression and therapeutic efficacy, this novel therapeutic concept needs further investigation using immunocompetent animal models. On the other hand, there is a need to develop non-inhalation CO delivery methods to allow for easy and controlled CO administration. Along this line, there have been intensive efforts in developing various CO delivery forms (19-25). Especially interesting to us is a group of organic CO prodrugs with tunable and controllable release properties (21,26,27). These organic CO prodrugs have been extensively studied for their pharmacological effects in models of kidney injury (26), liver injury (21,28), chemically induced gastritis (29), colitis (21), and general inflammation (28). Figure 2 shows some examples. Furthermore, the pharmacokinetic behaviors of some of these prodrugs have been extensively characterized (27,30). The application of these alternative delivery forms in studying CO’s effects on metastatic cancer prevention and treatment will hopefully lead to candidates for the clinical development of CO-based systemic cancer therapies.
Acknowledgments
We thank Cheryl Zhang for editorial assistance.
Funding: None.
Footnote
Conflicts of Interest: Both authors have completed the ICMJE uniform disclosure form (available at https://apc.amegroups.com/article/view/10.21037/apc-2022-4/coif). YCND is The Rasweiler Family Research Scholar in Cancer Research and received the following grants: NIH R01CA204916-01A1, DoD W81XWH-16-1-0619, and STARR I12-0043. YCND’s team has Utility Application filed on CO and metastasis. CO prodrug-related work in the lab of BW has been supported by the National Institutes of Health (DK119202 on CO and colitis; and DK128823 on CO and acute kidney injury), the Georgia Research Alliance Eminent Scholar endowment fund (BW), and internal resources at Georgia State University. BW’s team has several patents filed or approved on the CO prodrug work. The authors have no other conflicts of interest to declare.
Ethical Statement: The authors are accountable for all aspects of the work in ensuring that questions related to the accuracy or integrity of any part of the work are appropriately investigated and resolved.
Open Access Statement: This is an Open Access article distributed in accordance with the Creative Commons Attribution-NonCommercial-NoDerivs 4.0 International License (CC BY-NC-ND 4.0), which permits the non-commercial replication and distribution of the article with the strict proviso that no changes or edits are made and the original work is properly cited (including links to both the formal publication through the relevant DOI and the license). See: https://creativecommons.org/licenses/by-nc-nd/4.0/.
References
- National Cancer Institute SEER Program. Cancer Stat Facts: Pancreatic Cancer.
- Heye T, Zausig N, Klauss M, et al. CT diagnosis of recurrence after pancreatic cancer: is there a pattern? World J Gastroenterol 2011;17:1126-34. [Crossref] [PubMed]
- Yang X, Lu W, Hopper CP, et al. Nature’s marvels endowed in gaseous molecules I: Carbon monoxide and its physiological and therapeutic roles. Acta Pharm Sin B 2021;11:1434-45. [Crossref] [PubMed]
- Ryter SW, Ma KC, Choi AMK. Carbon monoxide in lung cell physiology and disease. Am J Physiol Cell Physiol 2018;314:C211-27. [Crossref] [PubMed]
- Szabo C. Gasotransmitters in cancer: from pathophysiology to experimental therapy. Nat Rev Drug Discov 2016;15:185-203. [Crossref] [PubMed]
- Wang B, Otterbein L. editors. Carbon Monoxide in Drug Discovery: Basics, Pharmacology, and Therapeutic Potential. 1st edition. Hoboken, NJ, USA: John Wiley and Sons, 2022.
- Rosas IO, Goldberg HJ, Collard HR, et al. A Phase II Clinical Trial of Low-Dose Inhaled Carbon Monoxide in Idiopathic Pulmonary Fibrosis. Chest 2018;153:94-104. [Crossref] [PubMed]
- Wegiel B, Gallo D, Csizmadia E, et al. Carbon monoxide expedites metabolic exhaustion to inhibit tumor growth. Cancer Res 2013;73:7009-21. [Crossref] [PubMed]
- Vítek L, Gbelcová H, Muchová L, et al. Antiproliferative effects of carbon monoxide on pancreatic cancer. Dig Liver Dis 2014;46:369-75. [Crossref] [PubMed]
- Zhang T, Zhang G, Chen X, et al. Low-dose carbon monoxide suppresses metastatic progression of disseminated cancer cells. Cancer Lett 2022;546:215831. [Crossref] [PubMed]
- Zhang G, Du YN. Orthotopic Pancreatic Tumor Mouse Models of Liver Metastasis. Methods Mol Biol 2019;1882:309-20. [Crossref] [PubMed]
- Pappalardo A, Giunta EF, Tirino G, et al. Adjuvant Treatment in Pancreatic Cancer: Shaping the Future of the Curative Setting. Front Oncol 2021;11:695627. [Crossref] [PubMed]
- Fiorito V, Chiabrando D, Petrillo S, et al. The Multifaceted Role of Heme in Cancer. Front Oncol 2019;9:1540. [Crossref] [PubMed]
- Canesin G, Di Ruscio A, Li M, et al. Scavenging of Labile Heme by Hemopexin Is a Key Checkpoint in Cancer Growth and Metastases. Cell Rep 2020;32:108181. [Crossref] [PubMed]
- O’Callaghan KM, Ayllon V, O’Keeffe J, et al. Heme-binding protein HRG-1 is induced by insulin-like growth factor I and associates with the vacuolar H+-ATPase to control endosomal pH and receptor trafficking. J Biol Chem 2010;285:381-91. [Crossref] [PubMed]
- Kwon YJ, Baek HS, Ye DJ, et al. CYP1B1 Enhances Cell Proliferation and Metastasis through Induction of EMT and Activation of Wnt/β-Catenin Signaling via Sp1 Upregulation. PLoS One 2016;11:e0151598. [Crossref] [PubMed]
- Abdul Aziz AA, Md Salleh MS, Mohamad I, et al. Single-nucleotide polymorphisms and mRNA expression of CYP1B1 influence treatment response in triple negative breast cancer patients undergoing chemotherapy. J Genet 2018;97:1185-94.
- Stine ZE, Walton ZE, Altman BJ, et al. MYC, Metabolism, and Cancer. Cancer Discov 2015;5:1024-39. [Crossref] [PubMed]
- Motterlini R, Otterbein LE. The therapeutic potential of carbon monoxide. Nat Rev Drug Discov 2010;9:728-43. [Crossref] [PubMed]
- Abeyrathna N, Washington K, Bashur C, et al. Nonmetallic carbon monoxide releasing molecules (CORMs). Org Biomol Chem 2017;15:8692-9. [Crossref] [PubMed]
- Ji X, Wang B. Strategies toward Organic Carbon Monoxide Prodrugs. Acc Chem Res 2018;51:1377-85. [Crossref] [PubMed]
- Reiländer S, Schmehl W, Popp K, et al. Oral Use of Therapeutic Carbon Monoxide for Anyone, Anywhere, and Anytime. ACS Biomater Sci Eng 2022; Epub ahead of print. [Crossref]
- Soboleva T, Berreau LM. 3-Hydroxyflavones and 3-Hydroxy-4-oxoquinolines as Carbon Monoxide-Releasing Molecules. Molecules 2019;24:1252. [Crossref] [PubMed]
- Johnston HM, Kueh JTB, Hartley RH, et al. Utilising fluorescent reporters to probe the mode of action of norbornen-7-one CO releasing molecules. Org Biomol Chem 2022;20:5812-9. [Crossref] [PubMed]
- Yang XX, Ke BW, Lu W, et al. CO as a therapeutic agent: discovery and delivery forms. Chin J Nat Med 2020;18:284-95. [Crossref] [PubMed]
- De La Cruz LK, Yang X, Menshikh A, et al. Adapting decarbonylation chemistry for the development of prodrugs capable of in vivo delivery of carbon monoxide utilizing sweeteners as carrier molecules. Chem Sci 2021;12:10649-54. [Crossref] [PubMed]
- Yang X, Lu W, Wang M, et al. Activated charcoal dispersion of carbon monoxide prodrugs for oral delivery of CO in a pill. Int J Pharm 2022;618:121650. [Crossref] [PubMed]
- Ji X, Pan Z, Li C, et al. Esterase-Sensitive and pH-Controlled Carbon Monoxide Prodrugs for Treating Systemic Inflammation. J Med Chem 2019;62:3163-8. [Crossref] [PubMed]
- Bakalarz D, Surmiak M, Yang X, et al. Organic carbon monoxide prodrug, BW-CO-111, in protection against chemically-induced gastric mucosal damage. Acta Pharm Sin B 2021;11:456-75. [Crossref] [PubMed]
- Wang M, Yang X, Pan Z, et al. Towards "CO in a pill": Pharmacokinetic studies of carbon monoxide prodrugs in mice. J Control Release 2020;327:174-85. [Crossref] [PubMed]
Cite this article as: Wang B, Du YCN. The anti-metastasis effect of low-dose carbon monoxide. Ann Pancreat Cancer 2023;6:1.